Dr. Areej Alhhazmi: Understanding the mechanism of actions of a heme binding antimicrobial peptide using a collection of different derivatives and a novel DNA-based strategy
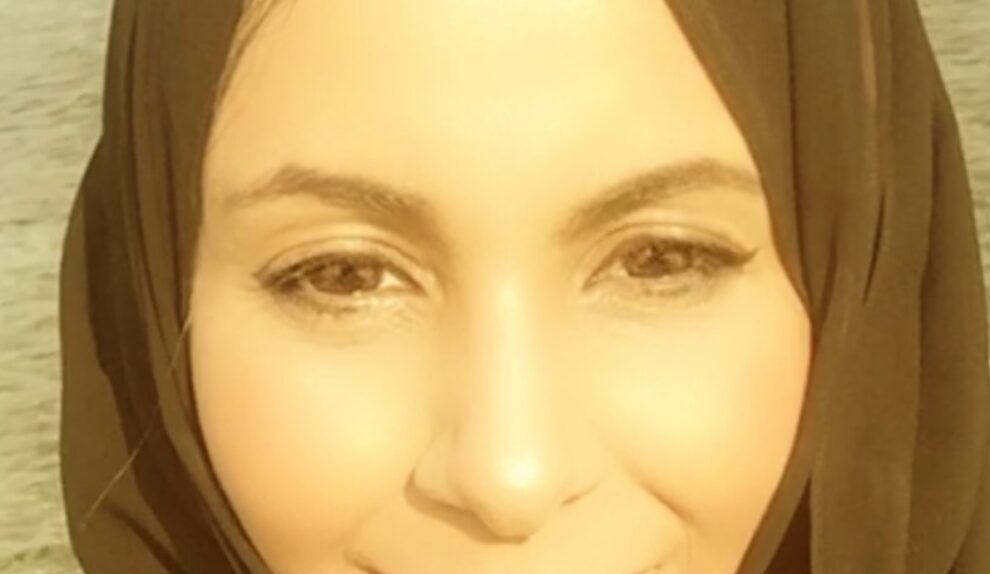
Dr. Areej Alhhazmi an Assistant Professor of Medical Bacteriology at Taibah University in Madinah, Saudi Arabia and an Ibn Khaldun Fellow in Professor Graham Walker’s Lab in MIT’s Biology Department.
Summary
One of Dr. Alhhamzi’s research interests lies in the area of tackling antibiotic resistance challenges. In particular, identifying and characterizing new antibacterial leads among natural resources, such as host defense peptides. In her research at MIT, Dr. Alhhazmi focuses on characterizing an iron sequestering/heme-binding plant peptide with antimicrobial activity. Dr. Alhhazmi performed an in-depth analysis of a collection of different variants of this peptide related to antimicrobial activity, mammalian cell toxicity, and iron-regulated gene expression. She has also developed an innovative regulated system in E. coli to dissect the membrane and sub-cellular actions of this peptide by specifically expressing it in different bacterial cell compartments. This strategy can be extended to study the mechanism of actions of other antimicrobial peptides (AMPs). Dr. Alhhazmi will be sharing the results of this study, providing a greater understanding of the mechanism of actions of multifunctional AMPs as the potential next-generation antibiotics.
WEBINAR TRANSCRIPT
DOROTHY HANNA: Good afternoon and good evening. My name is Dorothy Hanna, and I'm the program administrator for the KACST MIT Ibn Khaldun Fellowship for Saudi Arabian women. Welcome to today's IBK webinar, presented by Dr. Areej Alhhazmi based on her research here at MIT this year titled, "Understanding the Mechanism of Actions of a Heme-Binding Antimicrobial Peptide," fusing a collection of different derivatives and a novel DNA-based strategy.
After the talk, Dr. Areej will be answering questions, so please post your questions in the Q&A feature as we go along, and we will answer them at the end. To begin, our program director, Professor Kamal Youcef-Toumi, will introduce Dr. Areej's advisor, Professor Graham Walker. And thank you to everyone for being with us today.
KAMAL YOUCEF-TOUMI: Great. Yeah. Thank you, Dorothy, for the introduction, and I thank everyone for being with us today for Dr. Areej's webinar. I am happy to have everyone with us, and also very thankful to Professor Graham Walker, who has been supervising and working Areej's work. Professor Walker is a professor of biology at MIT. He has been working at MIT for over 40 years now. His work-- and notable work-- has been in explaining the structure and function of proteins involved in DNA repair, and the special applications have been in cancer and also understanding the effect in plants and mammals. So with this, I'll give the floor to Professor Walker to introduce Dr. Areej.
GRAHAM WALKER: Well, thank you very much, Kamal and Dorothy, for setting this up. It's a pleasure and an honor to actually introduce Areej, who is just a remarkable young scientist and incredible human being, as I'll try to give you just a tiny flavor of this as we go through.
Well, we're a long way from home at the moment. Areej got her BSC degree at King Abdulaziz University in Saudi Arabia. But then, she took a bold step and came to Canada-- I'm Canadian, actually. I grew up in Ottawa-- and did a master's degree in Saskatoon and Saskatchewan. And there, she worked on an aspect of bacterial DNA repair that was right on top-- an overlap with a lot of work I'd done in my career. But then, not minding the difference in climate, went to Edmonton, which-- even for Canadians-- is a cold place to be, and did her PhD there, where she picked up work on medical microbiology by focusing on an important aspect of some streptococcus isolates that cause invasive disease. And then moved from there back to Saudi Arabia, where she's an assistant professor of medical bacteriology at Taibah University in Medina.
And so, Areej entered my life when I got a call from this wonderful program saying, hm, we've got an interesting candidate. Maybe you'd like to meet her. And I looked at Areej's CV and it looked pretty interesting, so we set up a little Zoom talk. And my goodness, I'm going to say it was in about five minutes that I was dying to have her join my lab. We were on the phone for probably-- I don't know-- a long time, talking about this, talking about that.
So that all sounded wonderful, and Areej was-- I think there were 93 candidates. She was one of the five who was selected, so it was quite an honor, and we were both looking forward to her coming. But this is where I began to learn something about Areej as a person, and her resiliency and persistence. Because then, catching us both blindsided, her university decided they didn't really want to go right now, so she couldn't come and take this fellowship, which was a huge disappointment to Areej and to me, I must say. We conferred about this, and with help from MIT, too, I think-- and support-- she tried again. And this time, it worked. I wrote too, and somehow, it all worked out and she arrived-- I think it was-- was it on New Year's Day, I think, last year, back in what was another world at that time, I guess.
But she didn't make it easy for herself. She brought her-- she had her two sons, and her daughter was with her. And her husband was here for the first little bit getting settled in. And I guess, finally, with some concerns about health, it was decided it'd be probably better for her daughter to be in Saudi Arabia. But that means that Areej has not seen her daughter for a long time now, and is really looking forward to getting back. But she's been here with her two sons, who are 8 and 11, and not only doing all the stuff I'm going to tell you, but being basically a single parent. And that was challenging enough.
But then, in mid-March, came COVID and suddenly, the kids were at home and she couldn't go into the lab. And even when we could go into the lab, we ramped up to 25%, 50% and finally, up. But it's been-- she's shown such fortitude and such courage and just such cheerfulness throughout the whole thing. I'm so impressed. What a colleague to have.
She picked up-- we talked about possible projects and decided that the second area I work on, which is a symbiosis between a bacterium and a plant that you'll hear about, was a way of learning some key things about particular defense molecules called anti-microbial peptides that she'll tell you about. They've been adapted for a slightly different use in symbiosis, but you can get a lot of really fundamental research. And in spite of all the obstacles that I have talked about, she made some really major breakthroughs, and her contributions are going to be reflected in some papers that will be in the pipeline soon.
But this wasn't-- if this didn't sound challenging enough, she managed to take a bunch of courses over at Sloan and actually earned an MIT Management-- Executive Education Certificate from the Sloan School of Management. And part of this she managed to do during this complete shutdown. She was not only doing stuff related to her research, she was doing this. And I'm in awe, Areej. I don't know how you pulled it all off.
But you're just-- you've been a wonderful scientist to have in the lab. I'm incredibly excited by what you've done. I hope what you've learned will help you when you go back. You've been an amazing member of a research group, and especially as we all had to kind of support each other through this tough time, which has been terrific. You've been a great friend too, I must say, over that time. And it's just so wonderful to have had you with me. I'm going to miss you dearly-- desperately-- when you go, but it's great, and we're only a Zoom call away anyway. So anyway, I'll turn the floor over to you to see what you've brought to us.
KAMAL YOUCEF-TOUMI: Yeah.
AREEJ ALHHAZMI: OK. I'm going to share it now, so I think you can see my slides now, right?
GRAHAM WALKER: Yes, mm-hmm.
AREEJ ALHHAZMI: Yeah, great. So [NON-ENGLISH SPEECH]. It's such an honor and privilege to be amongst all of you here today. Thanks for taking time out of your busy schedule to attend this webinar, and I hope you will enjoy it and learn something out of it. And I want to say, at the beginning, I take pride and inspiration being a member of Professor Walker's lab. It's been amazing. Thank you, Professor Walker, for all the good words. Thank you for accepting me in your lab, actually. It's been a pleasure working with you and all the amazing scientists in your lab.
So before jumping to today's talk, I just want to share with you my agenda for today. So I'm going to start talking about one of the biggest challenges public health has been facing worldwide, which is antimicrobial resistance. And then, from there, I'm going to move to talk about how we can overcome this challenge. And specifically, talking about identifying new classes of anti-microbial agents. And then, from there, I'm going to talk about what I have been doing in the Walker lab, which is developing a new system to characterize the mechanism of these anti-bacterial agents. I used this method with other methods to characterize the mechanism of action of a specific and interesting peptide-- [INAUDIBLE] peptide-- with a promising antimicrobial activity. And finally, I'm going to share with you some of my post-IBK plans.
So to start up, antibiotics have saved many and many lives worldwide. But these drugs actually are losing their effectiveness because of antimicrobial resistance. A resistant infection can strike anyone-- children, seniors, healthy people, and sick people. And treating a resistant infection actually is very costly. It cost the US care system about $21-- up to $34-- billion annually. Many families have suffered the effects of antimicrobial-resistant infection, and indeed, some patients have lost their life due to these kinds of infection.
A very touching report published by Infectious Disease Society of America completing a heart-wrenching story about a family who lost one of their members due to this kind of infection. The name of the report is "The Faces of Antimicrobial Resistance." This report is part of the American Society initiative effort to seek global commitment to create antibiotic research and development enterprise to produce more systematic antibiotic or clinical therapy.
The path of antibiotic discovery actually-- from lab into clinical treatment or clinical setting-- has a high attrition rate. And you can sense that from this antibiotic discovery timeline [INAUDIBLE]. So to take you through it, all the green circles represent a timeline when an antibiotic has been discovered and has been used for treatment. And all the red circles represent a timeline when a pathogen has evolved some kind of resistant to the existent and discovered antibiotic.
Antibiotic discovery started in the '40s, with penicillin and streptomycin, and between the '50s and the '70s, more than 20 classes of antibiotics have been discovered. And this era is actually known as the Golden Era of antibiotic discovery. And not before the '90s, only two classes of antibiotics have been approved by the FDA for treatment.
A very striking story came out in 2016 about a female patient in her 70s diagnosed with a systematic inflammatory response, and then developed septic shock and died. And this patient actually was infected by a nasty bacteria that causes problems in the respiratory system. But actually, also was resistant to all 26 antibiotics that had been approved for her treatment in the US. So the antibiotic resistance has been increasing and spreading all over the world, and with the low base of antibiotic discovery development, this provokes some kind of urgency to address this issue. And one way to do that is by identifying new classes of antibiotics.
And one of these classes is antimicrobial peptide. It's a tiny protein produced by a living organism as one of the first line of defense against potential harmful encounters in the surroundings. These antimicrobial peptides-- AMPs-- actually exhibit a broad activity. It can kill bacteria, yeast, fungi, viruses, and even cancer cells. Speaking about the effect of antimicrobial peptides specifically on bacteria, these AMPs actually can interact with the cell surface of bacteria and create some pores in the cell surface, which lead to cellular leakage and eventually to cell death. And these AMPs were also found to be able to transport it inside the cell, interact with different targets inside the cell, and block some important processes, which can also lead to cell death.
Where we can find these AMPs? It can be produced and expressed by various living organisms-- mammals, insects, marine creatures, and even in our system as a human. [INAUDIBLE] Different kinds of AMPs can be produced either by our system or healthy bacteria that live in our gut. These AMPs actually, in nature, evolved to perform additional biological activity. And in the plant world, one example is a specific kind of peptide that actually important to establish a mutually beneficial relationship between soil bacteria, known as rhizobia, and a specific kind of plant. So the plant allows bacteria to invade the cell and live inside the plant cell, and the bacteria function as a fertilizer for the plant.
So what the bacteria do for the plant is converting atmospheric nitrogen, which is a form of nitrogen plants cannot be used into a more accessible form. And this process is known as a nitrogen fixation process. So specific kinds of plants allow soil bacteria, rhizobia, to invade the cell and live inside the plant root. And as a response, the plant develops a new organ, which is known as a nodule, where the soil bacteria can grow.
And in the nodule, the bacteria-- to be able to do the nitrogen-- or perform nitrogen fixation, it needs to go through some changes. And these changes meditate by plant peptides, produce in the nodule, and these peptides are actually known as a nodule-cysteine rich peptide. And some plants are able to produce more than 700 member of these peptides.
One representative of these peptides is NCR247, which has been studied in the Walker lab for many years. And it has a specific characteristic. It's one of the smallest peptides in NCR family, with only 24 amino acids. As you see in the top, there are some letters. These letters actually [INAUDIBLE] of amino acids that make up the NCR247 sequences.
Four of these amino acids are cysteine, highlighted in yellow. And this amino acids, cysteine, is able to form disulfide [INAUDIBLE] under the oxidized environment. Another interesting feature about NCR247 is that it has a high number of [INAUDIBLE] discharged amino acids, represented in the sequences by R, which is [INAUDIBLE], which make NCR247 a cationic peptide with a net charge equal to plus 6.
Another interesting feature about NCR247 is 29% of the amino acids that make up NCR247 are hydrophobic amino acids, which actually is amino acid that hates water. And it explains the ability of NCR247 to interact with multiple proteins. NCR247 resembles other AMPs in its antimicrobial activity.
So in a high concentration of NCR247, it exhibits antimicrobial activity against rhizobium, the soil bacteria, against plant pathogens, and also human pathogens. And one of the principal antimicrobial activities of this NCR247, like other AMPs, is interfering with the cell surface-- with the membrane of the bacteria by performing some kind of non-specific binding to the bacteria surface because it has a positive charge. And most of the bacteria cell surface actually has a negative charge, so this mediate the interaction-- the non-specific interaction with bacteria cell surface. And sometimes, spores can form and lead, as I said before, to cellular leakage and cell death. NCR247 also was found to be transported inside the cell and to interact with the multiBac protein, which can be explained, as I said before, because of the high hydrophobisity of NCR247.
Treatment of soil bacteria, rhizobium, with a low concentration of NCR247 was found to provoke a change in how the gene is expressed, and specifically, one group of genes that is responsible for importing iron into the bacteria cell, which is a very interesting observation about NCR247. Another clue is NCR247, from an evolutionary standpoint, is shares high similarity to the C terminus, or the end part, of heme [INAUDIBLE] protein and other bacteria. And heme, actually-- you may have heard this word associated with hemoglobin-- is one of the components of our red blood cells. It's a flat molecule with four rings, as you see, and in the middle, there is iron that can interact with oxygen and help in oxygen transportation and storage. And heme is very important for bacteria. It's a co-factor for many, many-- for multiple proteins, which is responsible for many enzymatic processes inside the cell.
So what's new about NCR247? A post-doc in Walker lab, Siva, was able to investigate the interesting observation about the NCR247, in terms of turning on an iron uptake gene, the gene responsible for taking more iron inside the cell, and also the similarity with the heme-binding protein in other bacteria. And she found a very interesting finding.
The first one is, actually, NCR247 binds heme with a high affinity. And also, in rhizobium, subinhibitory concentration treatment with NCR247 turns on the iron uptake gene. And this is because, in rhizobium, heme is responsible, actually, for regulating iron uptake genes. So once heme is present in the cell with a low concentration, it allows the bacteria to take more iron inside the cell. And once heme is, on the other hand, present in a high concentration, it prevents the cell from taking up more iron inside the cell. A very interesting finding of NCR247 is adding iron to where the bacteria grow. This cues the inhibitory effect of NCR247 in rhizobia.
So the proposed heme-binding model by NCR247 is-- inside the cell, heme can present either as a free heme with a low concentration and bound to other proteins, which actually functions as a co-factor for these proteins and allows these proteins to function. This is not what makes these proteins happy.
Once NCR247 gets inside the cell, it can bind to heme-- free heme-- and also can disturb heme from other proteins-- the proteins that bind heme. And this can block some very important processes, and in another way, also, binding NCR247 to heme can sequester iron and hide iron. And the bacteria set can think it's actually starving for iron, and that's why allowed the bacteria to take more iron inside the cell.
But heme-binding activity is not the only story for NCR247. NCR247, as I said before, resembles other AMPs. It can interfere with the membrane. It may have, also, other intracellular targets that can mediate the antimicrobial activity of NCR247. And unfortunately, the traditional way to study antimicrobial peptides has focused only on treating bacterial cells with synthetic peptides, and then doing the different kinds of assessment, either studying the effects of these peptides on the-- if these peptides can kill the bacteria or inhibit the growth of the bacteria, or shape the morphology of the bacteria or so on.
And also, these cannot distinguish-- these methods cannot distinguish between the effect of the peptide on the membrane and all the intracellular target of the bacteria. And this is interesting because, in bacteria, if we take E. coli as an example for a big group of gram-negative bacteria, we found the bacteria has a different compartment inside the cell. And to understand this, I'm going to briefly explain the bacterial cell structure in E. coli. So the bacteria actually covers with a cell wall in the blue line, which gives the cell-- the bacteria its rigidity. And then, after that, there is an outer membrane. And below that, we can find an inner membrane protein. And the space between an outer membrane and inner membrane is a periplasmic space with a different environment, different target to any drugs.
Inside the cell, or in the middle of the cell, there is the cytoplasmic, with the genetic material floating, and most of the-- and some of the enzymatic reactions happening inside the cells. So the bacteria cell actually consists of different compartments-- cytoplasmic, periplasmic, and also, we have the outer membrane common target for the antimicrobial peptide.
So to understand the effect of AMPs on different compartments of the cell, we cannot use the traditional method of studying these peptides. So here, I developed an innovative strategy and system to actually dissect the mechanism of action of AMPs on different compartments of the cell by allowing the expression of these antimicrobial peptides either in the outer membrane, in the periplasmic, or in the cytoplasma.
So the system I developed actually consists of three parts. The first part is an outer membrane protein projected from the C terminus into the external space. And the antimicrobial peptide links to the outer membrane protein by a tether. So it's holding the outer membrane through something like a rope.
And then, the second part is an inner membrane protein projected from the C terminus into the periplasmic space. And the antimicrobial peptide, actually, here also is linked to the inner membrane through a tether. The third part is inner membrane protein, but actually, here, it's projected from the C terminus into the [INAUDIBLE] cytoplasmic space. And the antimicrobial peptide, again, is linked to the inner membrane through a tether. And this system, actually, is regulated, which means when we grow the bacteria that have this system, the system is off. And after we add a specific kind of sugar, known as arabinose, the system can be turned on.
I tried the system with the three different peptides-- our NCR247 and a mutated version of NCR247, where all the cysteine is mutated into serine. And this version of NCR247 has shown lower antimicrobial activity and also cannot bind to home. The third peptide is a small peptide that does not have any effect on bacterial growth, and I use it as a control.
I constructed nine strains-- three strains for each compartment, for the outer membrane, the cytoplasmic, and the periplasmic space. And each compartment have three strains-- three peptides-- either NCR247, NSR247, or the negative control, HA. And speaking generally about the effect of antibiotic on bacteria, it has two effects. Either, it's stopping or limiting the bacterial growth. So once you take off the antibiotic, the bacteria can grow normally. And the other effect is killing the bacteria. So once you take the antibiotic, you see decrease in number of viable cells. And using the traditional way of studying peptides, synthetic NCR247, when treated-- when E. coli cell is treated with synthetic NCR247, it kills the bacteria at 6 micromolar after three hours of treatment.
So the first question I asked, what is the antimicrobial activity of the bacteria response I can see once I express NCR247 or the mutated version of NCR247 in different compartments of the cell? This slide is about the outer membrane compartment. So actually, we have the strain NCR247, NSR247, and the control in the outer membrane, and we grow it. I grow it for a couple of hours, and I added in some strain. I added arabinose to turn on the system, and other strain. On the other hand, the other strain was-- there was no arabinose, so the system was off. And I measured the growth of the bacteria for six hours.
I want to draw your attention, first, to the red stars here. So on the top, the red star is close to this line-- the orange line-- which represents the outer membrane that has NCR247. But the system is off, so we see a normal growth, or the bacteria is behaving normally. It's growing normally. And once we turn on the system, we see inhibition and bacterial growth after two hours, starting from two hours.
For NSR247, I want to draw your attention to the green circles. So the one on the top-- the yellow line-- it's NSR247 when the system is off, so it's behaving normally. And once it's turned on, actually, there was inhibition in the growth after two hours, like NCR247. But the inhibition is lowered-- is lower in NSR247. So this suggests that changing the cysteine in NCR247 actually is important, or cysteine is important for the interaction of NCR247 with the outer membrane of bacterial cells.
This slide is for the cytoplasmic compartment. I used the same strain-- I mean the same peptide, so NCR247, NSR247, and the control. And once the system is turned on in the cytoplasma, NCR247 shows inhibitory effect after one hour. However, NSR247 from the green-- close to the green star, grew normally, which is very interesting because NSR247 cannot bind heme, which suggests, actually, that NCR247, in the cytoplasma, the predominant role maybe is associated with the heme-bonding activity of NCR247.
In the periplasmic, once we express the NCR and NSR247, both show similar activity. The red one is the NCR247 and the green star is the NSR247, showing inhibitory activity after one hour, similar activity and similar pattern, which suggests, actually, maybe the activity we see here in the periplasmic is different than the one we saw in the cytoplasmic compartment.
And to summarize, NCR247 showed inhibitory activity on the three compartments. However, for NSR247, we only saw the inhibitory activity in the outer membrane and in the periplasmic, but not in the cytoplasmic. And then, I asked the question whether adding iron where the bacteria are growing and turning on the system can rescue the growth of bacteria in different compartments. So for expressing out NCR247 in the outer membrane and NSR247 in the outer membrane, adding iron actually did not change or rescue the growth of the bacteria. So we saw the same inhibitory activity in both. The blue line is with iron and the light blue is without iron. And it shows similar activity for NCR247 and NSR247.
But in the cytoplasmic, NCR247 showed different activity. So adding iron to the media actually rescued the growth of bacteria that have NCR247, but not the one that have NSR247, which doesn't bind to heme. So this is another piece of information suggesting that the primary role of NCR247 in the cytoplasma is maybe due to its to heme-binding activity.
Adding iron to the bacteria that have NCR and NSR247 in the periplasmic did not rescue the growth. And this is another piece of information suggesting also that the activity I'm seeing in the periplasmic is not due to-- is not similar to the one that I saw in the cytoplasmic compartment, which is-- we think it's mainly by the heme-binding activity of NCR247.
The inhibitory effect of NCR247 across the different compartments was different in terms of time. So NCR247 showed a faster activity in the periplasmic and the cytoplasmic within one hour. However, in the outer membrane compartment, it took two hours to see the inhibitory activity, which means, actually-- it would suggest, actually-- because the binding of-- or the activity of NCR247 in the outer membrane is not specific and needs more time to see the inhibitory activity.
And then I asked the question if the inhibition we see with NCR247, is it due to killing effect or static effect? So as I mentioned before, antibiotic either having a static effect, which limits the bacterial growth, and once you take off the effect of the antibiotic, you can see normal growth of the bacteria because the bacteria will be able to multiply. And a side effect is, once you take off the antibiotic, you see a decrease in number of viable cells. And I did it for NCR247, expressing it in different compartments of the cell-- in the outer membrane, cytoplasmic, and in the periplasmic-- and actually, as you see from the blue line, in the outer membrane, NCR247 has a more static effect, while in the periplasmic and the cytoplasmic, it's more a killing effect. We see a decrease in number of cells over time. For NSR247, similarly, expressing NSR247 on the outer membrane has a static effect on bacteria. But in the periplasmic, it has a more killing effect.
So to sum up this part, NCR247 possesses multifunctional activity at the membrane and the intracellular sites to achieve the efficient killing of NCR247 we saw in the-- when you use the traditional way of studying NCR247 effect on E. coli. And actually, the effect of heme-binding and the intercellularity inhibitory mechanism of NCR247 serve a very important goal contributing to the overall antibacterial efficiency of NCR247. As we saw in the cytoplasmic and the periplasmic, expressing NCR247 has a more killing effect than the outer membrane.
Another approach I used to study or characterize NCR247 is very interesting. Because NCR247 and antimicrobial peptides, generally, is the very small peptide, so you can manipulate sequences of these peptides and synthesize different variants of these peptides. And you can actually study structural functional activity by doing that with different derivatives.
And this is what I did with NCR247. So a library with 24 different variants of NCR247 was synthesized with a shorter fragment of NCR247, having only the first 12 amino acids, or the last 12 amino acids. And a different other bunch of mutations in NCR247 that increase the net charge or decrease the net charge of NCR247, or have the same net charge as the wild type, which is the original type of NCR247. But different mutation in specific site.
Here, the number is only for labeling purposes, and N is for the first amino acid, the NCR fragment that has only the first 12 amino acids. And the C here is for the last-- for the fragment that has only the last 12 amino acids. So we have this NCR247 with very promising antimicrobial activity, heme-binding activity. It has a multifunctional activity on different compartments in the cell.
So I asked the question, if we can use this as a [INAUDIBLE] to use this for theoretical application, we need to understand more about if this peptide exhibits some kind of toxicity to human cells. So I did two toxicity assays. The first assay, I used a human red blood cell as an indicator of NCR247 toxicity toward mammalian cells. So red blood cells were treated with the NCR247 in the wild form, or the different variants. And then I measured the lyses-- the ability of these peptides to lyse the red blood cell.
The last-- or the one that's highlighted with red-- actually is the wild form, a wild type of NCR247. And interestingly, these peptides-- the wild form of NCR247-- did not show any toxicity towards red blood cells. However, other variants showed variation of toxicity above the 20% threshold.
Another toxicity assay I have done is using a HEK293 cell line, which is embryonic kidney cell line that's commonly used to study the genotoxicity and the cytotoxicity in drug discovery. I treated the cell with NCR247 in the wild form and the different variants. And then, after 24 hours, I measured the number of viable cells and calculated the toxicity percentage. Again, NCR247 in the wild form did not show any toxicity toward this cell line. Other variants, though, show some toxicity above the 20% threshold. So NCR247, here, we have it with a promising antimicrobial activity, with a multifunctional activity, with a very low toxicity profile to present itself with multiple critical applications.
And the final thought for today is during my time in the Walker lab, I was able to develop a tether system. And this system is the first approach to dissect the mechanism of action of antimicrobial peptides. It's a more general approach that can be adopted to study various antimicrobial peptides. And this tether system actually provided us with insightful data related to NCR247, this promising peptide for therapeutic application. It provides us with very insightful data related to the mode of action of this peptide.
Another approach I used is manipulating the sequences of NCR247 to study the-- or characterize the mechanism of action of NCR247. This approach also is general. It can be generalized to study the mechanism of action of various peptides.
So some of my plans, post-IBK, is one of the projects I'm working on is using the tether system and the other approach I used for characterizing NCR247 with a different member of NCR peptide. And another project-- I'm collaborating with a group of smart and intelligent computer science and engineering scientists. We were inspired by COVID, and we formed a team to develop an algorithm to study or to predict the next pandemic with the aim to get at the end product of interface based on this model.
Another thing I'm working on, collaborating with two amazing scientists from Taibah University, Dr. Firdose and Dr. Nadia And also with an ambitious surgeon from King Faisal Specialist Hospital to identify a microbial marker for colorectal cancer patients to replace the invasive and the common way to screen the population for colorectal cancer. Another big plan for me is I'm very passionate about teaching, and I'm happy to get back to my class with my students and start teaching again once I get back to Saudi Arabia.
And I just want to say, at the end, IBK slash MIT journey wasn't a straight line road for me. It has some very complicated moments. It has tangled moments. It has complex loops. It has ups and down. I have faced many challenges. It wasn't easy for me. It's hard work, actually. And specifically during this COVID-19. And many people have told me that I joined MIT in the worst time, but actually, I like to look at-- or turn this negative experience to a positive experience. And I'm a very strong believer, so I think all the crucible moment and all the experience that I have been through this year helped me grow personally and leverage my skill and my knowledge, arm me with a new perspective, a new understanding. And I'm sure it's going to be handy in the future.
And actually, I couldn't have done any of the work without the help of the great people in my life-- my family back home, my two boys here, and Walker lab. The warmth and the kindness I have experienced from the very first day has touched me and has amazed me, actually. I couldn't have done anything without the help of Professor Walker, Siva, Vignesh, Charlie, Marcus, Kevin, Prine, and Mary. Thank you so much for your help and for being great scientists, and also great friends for me.
Also, my genuine thanks go to IBK team-- Professor Kamal, Theresa, Nadiyah, and Dorothy. Without your help, without your support, maybe I wouldn't be with you today. Thank you so much and thank you for your attention, for your listening for all the audience. And I'm very happy to answer all of your questions. Thank you.
PRESENTER: Thank you so much for sharing your research with us today, Areej. We appreciate you. As I was listening, I kept thinking, heroes don't always wear capes. So thank you for helping to make the world healthier and safer in our future. We are open for questions, so please use the question-and-answer feature. Let us know what you're wondering about, what questions you have for Dr. Areej, and we will give her a chance to answer them. Yes, Professor Kamal, go ahead.
KAMAL YOUCEF-TOUMI: Yeah, sorry. Maybe it will be just easy for me to say that-- yeah, thank you, Areej, for this great presentation. This is way far from what I do. I think the closest work that we have done to yours was in DNA sequencing in building, actually, instruments that do the sequencing at the nanoscale and looking at one molecule or one nucleotide-- one base-- at a time. And also, or some of our work deals with the visualization, to look at the nanoscale and see how things are happening.
So I was curious whether-- actually, to you and also to Graham-- whether there are any things here that need, or can be or would be desirable to see at the nanoscale. How are these things happening? Because this is what we can see with our high-speed imaging that we do in our lab. Imagine you can see, maybe if it's something dying-- that process of dying, for example, that you could see. Or if there is an interaction between different elements that you can see it dynamically as it happens. So this is what we can do with our instruments, and I'm curious to hear from you guys on whether you have-- there is such a thing in these elements.
AREEJ ALHHAZMI: Actually, what's interesting about this tether system, it's actually [INAUDIBLE] the activity of antimicrobial peptides in different compartments. And actually, we can study the morphology of the expressing [INAUDIBLE] peptide once it's expressed in different compartments. And one other thing I didn't share with you is a study is using the fluorescent microscopy to study the effect of expressing, for example, NCR on the outer membrane, and seeing if it can change the structure of the-- the morphology of the bacteria. As bacterial morphology is changing, is it, for example, getting [INAUDIBLE] indication of any change or disformation on the surface of the bacteria because of these peptides?
But electron microscope has also been used to study these peptides using the traditional method. But it would be very interesting to use all the available technology-- electron microscopy, [INAUDIBLE] microscopy, and all the downstream applications to study the effect of these peptides on bacterial cells. And Graham, I'm sure you have amazing ideas you can share.
GRAHAM WALKER: Well, maybe-- thank you for the offer, and maybe it's worth a follow-up chat. Let me float just another one off the top my head. I'm going to fill in something that Areej didn't have time to really get to in her talk about this NCR peptide. The way [INAUDIBLE] actually found it was we were interested in trying to put stable isotopes into-- we wanted to be able to express it in the cell so we could grow it and then cleave it. So we fused it to a protein-- maltose binding protein is widely used as a carrier protein, but there's a little tiny peptide at the back, and we were planning to cleave it off later. But when they purified this maltose binding protein with a little bit of the NCR247 peptide on it, it was brownish-red, and that was the initial clue that, actually, something was binding there. And Siva worked out the chemistry very convincingly. It was heme.
But because it happened to be fused to this maltose binding protein, Vignesh, who did the protein purification, put it over a sizing column, and we were startled to find that not only did bind heme, but it subsequently multimerized. And at least when it's fused to this very large-- relatively large maltose binding protein, it formed a dodecamer. And so we accidentally discovered this thing did two things-- the peptide binds the heme, and we think the stoichiometry probably means that there's a sandwich or something with one peptide on each side of that heme molecule. And then it subsequently multimerizes in some way. In some preliminary results that Siva has, where you would take the free peptide and add heme, it somehow forms multimers fairly quickly. But if you can do things at the single molecule level, and you can look at them really fast, we've got versions of that peptide that have a fluorescent label on them.
[INTERPOSING VOICES]
Wonder if it might be worth a quick little look. [LAUGHS]
KAMAL YOUCEF-TOUMI: Yeah, yeah. Yeah. Yeah, so the thing with this is that if the process is slow in any one of these applications-- so taking images by any of the conventional instruments is fine because things are not changing that fast. But if you have any kind of a process that might happen at a faster rate, it would be maybe useful to see exactly what is happening from the beginning of that process until the end of it. And then to figure-- maybe to have more information throughout the evolution of the process itself, whether it's binding or changing form or color or corrugation maybe on the surface that is how it is happening. So it could be-- yeah.
GRAHAM WALKER: Well, let's talk about it. I think, when we do just the pure peptide and the heme, it's happening faster than anything we can measure in our lab [INAUDIBLE]. So it might be worth a chat.
KAMAL YOUCEF-TOUMI: Sure, yeah. I look forward to it, Graham.
GRAHAM WALKER: OK.
AREEJ ALHHAZMI: Amazing.
KAMAL YOUCEF-TOUMI: Yeah.
DOROTHY HANNA: That's exciting to be able to make a connection there. It doesn't seem that there are any questions coming in from attendees. So it means that you were very thorough, Areej, in explaining everything, and very clear.
KAMAL YOUCEF-TOUMI: Yes.
PRESENTER: So thank you again for being here. Thank you to all of our attendees.
KAMAL YOUCEF-TOUMI: Thank you, everyone. Yeah.
PRESENTER: Yeah. Thank you.
KAMAL YOUCEF-TOUMI: Thank you, [INAUDIBLE]. Thank you, Graham, for being with us today.
GRAHAM WALKER: Thank you.
KAMAL YOUCEF-TOUMI: This was great. Yeah.
GRAHAM WALKER: Could I add my compliments? That was a lovely talk. You've put a lot of work into that, and that was-- oh, boy, it really showed the work you did. I thought it was really terrific-- a terrific talk. And I hope people gathered it was exciting. Great.
KAMAL YOUCEF-TOUMI: Excellent.
PRESENTER: Agreed.
KAMAL YOUCEF-TOUMI: Excellent, yeah.
PRESENTER: Great job, Areej. We're so proud of you. Thanks for everything.
KAMAL YOUCEF-TOUMI: Thank you.
GRAHAM WALKER: Theresa, thanks for hanging in with me through the--
AUDIENCE: Always. It's nice to see you.
[LAUGHTER]
It's been a long journey, but I'm so happy to see these great results. So I hope you're both very proud of this great accomplishment.
GRAHAM WALKER: Yeah. She's really pried open a new way to do things, and maybe you can get a sense of that.
AUDIENCE: Yeah.
KAMAL YOUCEF-TOUMI: Yes.
GRAHAM WALKER: Just added stuff to cells and now you can kind of put it in different parts of the cells and see what happens. So I think that's good.
AUDIENCE: Definitely.
GRAHAM WALKER: Yeah.
AUDIENCE: Yeah. Well, have a good day, good evening, depending on where everyone's logging in from.
KAMAL YOUCEF-TOUMI: Yes.
AUDIENCE: This has been great. Thank you.
KAMAL YOUCEF-TOUMI: Excellent. Yeah. Thank you, everyone. Thank you, Areej and Graham.
GRAHAM WALKER: Thanks again.
PRESENTER: Good night.
KAMAL YOUCEF-TOUMI: Dorothy and Theresa, thank you again. Yeah. Take care, everyone.
PRESENTER: Goodbye.
KAMAL YOUCEF-TOUMI: Yeah. Bye-bye.
GRAHAM WALKER: Bye.
Webinar Date: December 2, 2020 2pm EST/ 10pm AST